http://nextbigfuture.com/
August 22, 2015
The European Union SR2S project not only investigates the principles and the scientific problems (of magnetic shielding), but it also faces the complex issues in engineering.
Superconducting magnets, commonly found in MRI scanners, produce stronger, more efficient magnetic fields using smaller and lighter magnets than magnets made using conventional materials such as copper or aluminium.
On earth, superconducting materials must be cooled to very low temperatures using liquid helium to utilize their superconducting properties, however the project has already found a solution that will work in space.
‘We have decided to use a new superconducting material, discovered in 2001: magnesium diboride or MgB2,’ explained Dr Musenich. MgB2 can superconduct at 10 kelvin, or -263 degrees Celsius, which removes the need for liquid-helium cooling as this temperature is comparable with that of deep space.
Simulations of the magnetic system suggest that a 10-meter-diameter magnetic field could be produced by a system weighing less than half that of a comparable passive shield.
The SR2S superconducting shield will provide an intense magnetic field, 3,000 times stronger than the Earth’s magnetic field and will be confined around the space craft.
An active deflector shield system could never replace passive shielding or biological advances, but it can offer options, particularly for EVAs, extending the longevity of hardware and preventing secondary activation of the ship's hull and systems. It seems the only credible theory for deflection of GeV particles.
CERN will be helping to test the superconducting radiation shield design
NASA has a 158 page report on superconducting radiation shielding. Magnetic fields with 10 tesla and 10 meters thickness could deflect about 93% of the radiation. The EU design has overall protection against 50-70% of the radiation.
A superconducting shield to protect astronauts
The CERN Superconductors team in
the Technology department is involved in the European Space Radiation
Superconducting Shield (SR2S) project, which aims to demonstrate the
feasibility of using superconducting magnetic shielding technology to
protect astronauts from cosmic radiation in the space environment. The
material that will be used in the superconductor coils on which the
project is working is magnesium diboride (MgB2), the same type of conductor developed in the form of wire for CERN for the LHC High Luminosity Cold Powering project.
“In the framework of the project, we will test, in the coming months, a racetrack coil wound with an MgB2 superconducting tape,” says Bernardo Bordini, coordinator of CERN activity in the framework of the SR2S project. “The prototype coil is designed to quantify the effectiveness of the superconducting magnetic shielding technology.”
During long-duration trips in space and in the absence of the magnetosphere that protects people living on Earth, astronauts are bombarded with high-energy cosmic rays that might cause a significant increase in the probability of various types of cancers. Because of this, exploration missions to Mars or other distant destinations will only become realistically possible if an effective solution for adequately shielding astronauts is found. “If the prototype coil we will be testing produces successful results, we will have contributed important information to the feasibility of the superconducting magnetic shield,” says Ballarino.
There are many more challenges to overcome before a spacecraft shield can be built: various possible magnetic configurations need to be tested and compared and other key enabling technologies need to be developed. But the MgB2 superconductor seems to be very well-placed to take part in this challenging adventure as, among its many advantages, there is also its ability to operate at higher temperatures (up to about 25 K) thus allowing the spacecraft to have a simplified cryogenic system. Watch this “space”!
The SR2S Project
SR2S
is a Collaborative Project under the Space Theme of the EU Seventh
Framework Research Programme. The project started in January 2013 and
will end in December 2015. The aim of the SR2S project is to provide a
pathway for the further development of a protective shield for
astronauts, which should be launched in the market within the next two
decades.
Radiation Protection and Architecture Utilizing High Temperature Superconducting Magnets
NASA Johnson Space Center

› Larger photo
› Phase I Final Report (PDF)
NASA Technical Paper with update in work
Human space exploration exposes astronauts to particularly hazardous environments unique from Earth-based hazards. A substantial risk for exploration beyond the confines of the Earth’s geomagnetic field is radiation exposure from energetic solar protons and Galactic Cosmic Radiation. The concept of shielding astronauts with magnetic/electric fields has been studied for over 40 years and has remained an intractable engineering problem. Superconducting magnet technology has made great strides in the last decade. Coupling maturing technology with potential innovative magnet configurations, this proposal aims to revisit the concept of active magnetic shielding. The focus of the proposed work is to analyze new coil configurations with current technology and compare shielding performance and design mass with alternate passive shielding methods.
Space Radiation Superconducting Shield
Protection against radiation in space
is one of the foremost problems in human deep space exploration. As
governments and private enterprises are gearing towards human
exploration beyond Earth orbit, the issue of space radiation has taken
center stage. Beyond the shield of the Earth’s magnetosphere, astronauts
in deep space will be exposed to high energy Galactic Cosmic Rays (GCR)
and lower energy Solar Energetic Particles (SEP), both of which increase the chance of cancer, affect the central nervous system and might cause other degenerative diseases. So, any future human travel in deep space needs to solve this problem to be feasible.
– Space Radiation
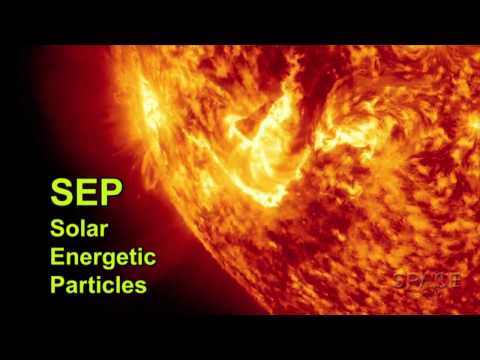
Several space agencies and research
institutions around the world are conducting research into spacecraft
shielding technologies. These can be categorized into two basic types.
Passive shielding involves the use of some material that will absorb
incoming radiation. But they become impractically massive for shielding
against GCRs and would also break up the GCR into harmful secondary
radiation. Active shielding uses electric or magnetic fields to deflect radiation and would be effective against both GCR and SEP.
Some of the leading work in active
radiation shielding is being undertaken by the Space Radiation
Superconducting Shield (SR2S). It is an EU-funded project whose aim is
“to develop, validate and increase the Technology Readiness Level (TRL)
of the most critical technologies related to a magnetic shielding system for protecting astronauts’ lives during
long duration space missions.” The project, started in January 2013,
has received funding under the EU Seventh Framework Research Programme –
Space Theme and will run until December 2015. The participating
organizations include Italian Institute for Nuclear Physics (INFN),
Compagnia Generale per lo Spazio (CGS SpA), Columbus Superconductors,
Thales Alenia Space – Italia S.p.A. (TAS-I), Commissariat à l’énergie
atomique et aux énergies alternatives (CEA), CERN, and Carr
Communications.
The project is developing a shielding
system powered by a superconducting magnet that will deflect radiation,
similar to the Earth’s magnetosphere. Superconducting magnets generate
strong magnetic fields compared to traditional magnetic materials. But
superconductors work only at extremely low temperatures. SR2S plans to
overcome this problem by using a new superconductor, magnesium diboride MgB2, which can operate at -263 Celsius and thus doesn’t require liquid helium cooling.
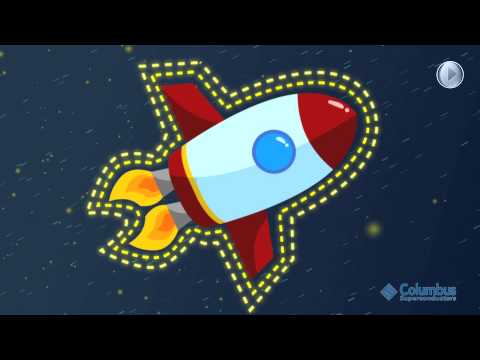
The SR2S project has conducted a study
on the feasibility of a superconducting magnetic shield, comparing the
various possible magnetic configurations and analyzing its merits as
well the challenges of this approach. It has also developed some key
enabling technologies required to build such a spacecraft shield. Other
major milestones include an assessment of the requirements for a super
conducting magnetic radiation shield and a realistic configuration to be
used in the short term to design demonstration units. Project partners
have also conducted review, analysis and selection of the requirements
on radiation doses for exploration travel in deep space.
Professor Roberto Battiston
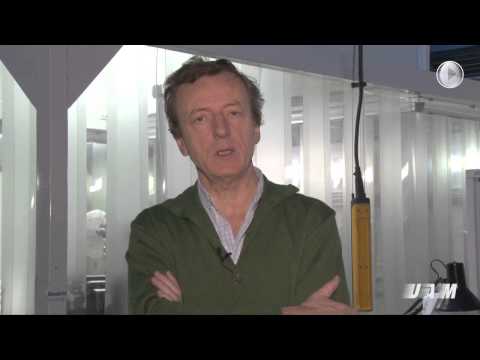
For more information, please visit the project website at http://www.sr2s.eu/
Radiation shielding to protect a mission to Mars
21 July 2014

The EU-funded SR2S project is developing magnetic
shielding that can deflect dangerous cosmic rays. Image: SR2S
Lightweight
magnetic shields could be the best way to protect an astronaut from
deadly radiation as they travel to Mars or beyond.
Long-term exposure to galactic cosmic rays and solar particles can lead to a significantly higher risk of developing cancer, researchers believe.
Increasing the thickness of spacecraft walls would be enough to protect astronauts from low-energy particles from the sun, however high-energy galactic cosmic rays would interact with the shielding materials to produce even more radiation.
The EU-funded SR2S project is developing magnetic shielding instead that can deflect these dangerous cosmic rays in the same way as the earth’s magnetic shield protects humans from cosmic radiation.
The idea, originally proposed in 1969 by space engineer Wernher von Braun, the so-called father of rocket science, is to use a superconducting magnet to create the shield.
‘As the magnetosphere deflects cosmic rays directed toward the earth, the magnetic field generated by a superconducting magnet surrounding the spacecraft would protect the crew,’ said Dr Riccardo Musenich, scientific and technical manager for the project.
‘SR2S is the first project which not only investigates the principles and the scientific problems (of magnetic shielding), but it also faces the complex issues in engineering.’
Dr Riccardo Musenich, scientific and technical manager, SR2S
Superconducting magnets, commonly found in MRI scanners, produce stronger, more efficient magnetic fields using smaller and lighter magnets than magnets made using conventional materials such as copper or aluminium.
On earth, superconducting materials must be cooled to very low temperatures using liquid helium to utilize their superconducting properties, however the project has already found a solution that will work in space.
‘We have decided to use a new superconducting material, discovered in 2001: magnesium diboride or MgB2,’ explained Dr Musenich. MgB2 can superconduct at 10 kelvin, or -263 degrees Celsius, which removes the need for liquid-helium cooling as this temperature is comparable with that of deep space.
Simulations of the magnetic system suggest that a 10-metre-diameter magnetic field could be produced by a system weighing less than half that of a comparable passive shield.
Habitats for extreme environments
Once the astronauts have arrived on Mars with both themselves and their spacecraft intact, the thin atmosphere and weak magnetosphere means they still won’t be safe from harmful radiation. Researchers on the SHEE project are attempting to reduce the economic and human cost of establishing outposts in the most inhospitable environments.By identifying common architectural considerations for extreme environments on earth and in space, the project will develop a self-deployable living and working space, which includes a rigid section to house life support systems, folding sections for workspaces and robotic motors to aid with deployment.
Future versions of the SHEE habitat could also be used on earth, providing shelters in areas recently hit by natural disasters, or mobile laboratories in difficult-to-access environments. Initial construction of a prototype began in April 2014 and a fully operational habitat suitable for two people will be finished in 2016.
For details, visit http://www.shee.eu/main
Shields up for manned space exploration
Artistic representations of an active, magnetic, toroidal shield used for protecting astronauts from
astroparticles during the transfer in orbit
astroparticles during the transfer in orbit
The project’s advances include cutting-edge superconducting materials, a novel approach to avoiding potentially destructive heat build-up in the magnets, and a powerful system to cool the equipment on the side exposed to the sun.
These breakthroughs are not just important for the future of manned space exploration. They also stimulate innovation here on Earth. Superconductors underpin technologies as diverse as magnetic resonance imaging scanners and particle accelerators.
Deflecting radiation with magnets
The powerful ionising radiation in space is one of the main issues to be tackled if humanity wants to head out into the starry void, says project coordinator Roberto Battiston of Italy’s National Institute for Nuclear Physics, who was appointed president of the Italian Space Agency in May 2014. On Earth, we are sheltered — the atmosphere provides radiation shielding equivalent to 3.5 metres of aluminium, in addition to the protection offered by our planet’s shadow and magnetic field.
“Astronauts in deep space will receive a hundred times more radiation than on Earth,” Battiston remarks. “This has not so far been a major limitation for space exploration, because currently astronauts don’t stay in deep space very long.” A return journey to Mars, which would involve approximately one year in deep space, would be a different matter entirely.
The concept of a magnetic shield is not new, says Battiston, but prior to SR2S it had never been explored in detail. “This is the first programme set up to tackle the various challenges of an active shield for space,” he explains. “One possible approach is to build a magnet that creates a field able to sweep the particles before they get into the craft. Obviously that’s a challenge, but at the moment it’s the most promising idea we have. SR2S is improving the technologies that are needed for this idea to become a reality.”
Superconductors offer no electrical resistance to electrical currents running through them, meaning these currents can be maintained without access to a power source — an important consideration for applications in space. That said, materials with superconducting properties only display these at very low temperatures, which means that the magnets have to be cooled.
Superconducting magnets are, of course, already widely used. However, at the moment they are too large and too heavy to be of practical use in space flight. SR2S has designed lighter, smaller components and systems based on magnesium diboride, which offers the added advantage of being far less costly than other superconducting materials.
The project has also developed the systems needed to keep these magnets running: powerful cryogenics, an innovative system to dissipate excess energy during transitions from superconductivity to normal conductivity, and an array of light, small pipes that will move heat away from the part of the hull that is exposed to the sun, towards the craft’s cooler side.
Mars matters
The project will end in December 2015, but further development is needed to prepare the technology for deployment, says Battiston. He expects this final stage to take 10 to 20 years.
And then, in terms of the radiation shielding, it’s all systems go for human exploration of our planetary neighbourhood. Without it, that’s not an option, according to Battiston. “You can’t send people to Mars just to have them die of cancer when they return to Earth,” he says.
SR2S is thus helping to ensure that we will eventually be able to boldly go where no Earthling had ventured before. “Tackling such a difficult problem is fascinating,” Battiston concludes. “It calls for new ideas and new approaches to push the technologies to the extreme. This kind of challenge is an extraordinary technology driver, which first and foremost benefits applications on Earth.”
Below artistic representations of an active, magnetic, toroidal shield used for protecting astronauts from astroparticles during the transfer in orbit viewed from the top (fig.2) and viewed in perspective (fig.3)
© SR2S / Image: Giorgina Colleoni & Valerio Calvelli
Imagining and Planning Interstellar Exploration
Braking Against a Stellar Wind
This morning I want to pick up on the ‘problem of arrival’ theme I
began writing about on Friday, and we’ll look at interstellar
deceleration issues a good bit this week. But I can’t let Monday start
without reference to the Icarus results from Gran Sasso that finds
neutrinos traveling at precisely the speed of light. All of this adds
credence to the growing belief that the earlier Opera experiment was
compromised by equipment problems. The news is all over the place (you
might begin with this BBC account)
and while we’ll keep an eye on it, I don’t plan to spend much time this
week on neutrinos. We still have much to get done on the subject of
slowing down.
Magsails and Local Resources
When you begin to unlock the deceleration issue, the options quickly multiply, and you find yourself looking into areas that weren’t remotely the subject of your earlier research. As we saw on Friday, the concept of magnetic sails grew organically out of Robert Bussard’s idea of an interstellar ramjet. Bussard didn’t want to slow down — he wanted to go very fast indeed. Read the comments on that post and you’ll find Al Jackson’s entertaining reminiscences of a dinner with Bussard (Tau Zero author Poul Anderson was present too), and a reminder that the scientist always claimed to have come upon the ramjet idea because of an encounter with Mexican food. The usual story has it that it was a burrito which, bitten down upon, suddenly opened for Bussard the splendors of matter being forced into a cylinder at high speeds.
Or maybe he was eating huevos rancheros — the story seems to have varied a bit over the years. Whatever the case, the idea of scooping up interstellar hydrogen and fusing it turned into a 1960 paper for Acta Astronautica and, along the way, into a critique by Robert Zubrin and Dana Andrews that showed just how much drag an electromagnetic scoop could generate. Andrews was working for Boeing at the time, and had grown interested in using Bussard concepts right here in the Solar System, thinking that a big enough scoop could gather hydrogen for use in an ion engine that could be powered up by an onboard nuclear reactor. A self-fueling ion drive might not be adaptable for interstellar missions, but for interplanetary work it seemed worth a look.
But the numbers were intractable. The magnetic scoop Andrews hoped to deploy created more drag than the ion engines produced thrust. The two researchers quickly found that the scoop’s best function was as a magnetic sail, and their work on the idea appeared in the literature in the early 1990s. In his 1999 book Entering Space, Zubrin recalls that the time was right for the magsail given that Paul Chu (University of Houston) had just invented the first high-temperature superconductors, which a magsail could theoretically use to create the magnetic field that would allow it to ride on the solar wind. Practical high-temperature superconducting wire born out of this work might one day allow magsails to achieve higher thrust-to-weight ratios than solar sails.
Magsails have clear propulsion implications, but Zubrin states the obvious about their most effective uses:
Rise of the Superconductor
When Bussard studied how his ramjet could operate in a region of interstellar space where the density of hydrogen was roughly 1 hydrogen atom per cubic centimeter, he saw that he would need a collecting area of 10,000 square kilometers. This is so vast that even if it were made of 0.1-centimeter mylar, a physical scoop would weigh something on the order of 250,000 tons. But a much smaller collector generating a magnetic field seems practical given the advances in superconducting alluded to above, with a loop of superconducting wire deployed from the spacecraft, the current applied to it cycling continuously to generate the magnetic field. Here’s how Zubrin and Andrews described it in a paper based on their presentation at the 1990 Vision-21 symposium at NASA’s Lewis Research Center (now Glenn Research Center):
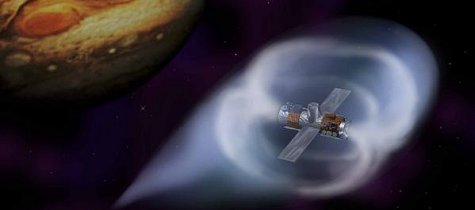
Image: A space probe surrounded by a magnetic sail. Early work
on these concepts has taken place at the University of Washington
under Robert Winglee, with reports available at NASA’s Institute
for Advanced Concepts site. Credit: NASA/University of Washington.
Thus the hybrid concept Andrews and Zubrin came up with in the Vision-21 work, extending ideas they had first presented in a 1988 paper: Use laser beaming technology to push a sail to interstellar cruise speeds, then deploy a magsail upon arrival to reduce deceleration time. The authors looked at the numbers and worked out 0.8 years for acceleration, 17.4 years of coasting at almost half the speed of light, and 18.8 years for deceleration. This gets you about 10 light years out in around 37 years, a mind-bending pace that uses a huge sail and some generous assumptions about laser power that we’ll look at tomorrow. For there are other ways to use lasers, even for deceleration, and other ways, too, to exploit the local interstellar medium.
Zubrin and Andrews’ paper from Vision-21 is “Use of Magnetic Sails for Advanced Exploration Missions,” in the proceedings of Vision-21: Space Travel for the Next Millennium” (NASA Conference Publication 10059. The citation for their 1988 work is given in yesterday’s Centauri Dreams post.
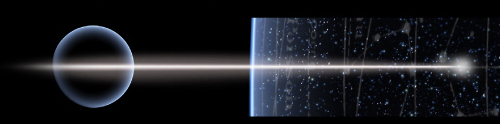
Magsails and Local Resources
When you begin to unlock the deceleration issue, the options quickly multiply, and you find yourself looking into areas that weren’t remotely the subject of your earlier research. As we saw on Friday, the concept of magnetic sails grew organically out of Robert Bussard’s idea of an interstellar ramjet. Bussard didn’t want to slow down — he wanted to go very fast indeed. Read the comments on that post and you’ll find Al Jackson’s entertaining reminiscences of a dinner with Bussard (Tau Zero author Poul Anderson was present too), and a reminder that the scientist always claimed to have come upon the ramjet idea because of an encounter with Mexican food. The usual story has it that it was a burrito which, bitten down upon, suddenly opened for Bussard the splendors of matter being forced into a cylinder at high speeds.
Or maybe he was eating huevos rancheros — the story seems to have varied a bit over the years. Whatever the case, the idea of scooping up interstellar hydrogen and fusing it turned into a 1960 paper for Acta Astronautica and, along the way, into a critique by Robert Zubrin and Dana Andrews that showed just how much drag an electromagnetic scoop could generate. Andrews was working for Boeing at the time, and had grown interested in using Bussard concepts right here in the Solar System, thinking that a big enough scoop could gather hydrogen for use in an ion engine that could be powered up by an onboard nuclear reactor. A self-fueling ion drive might not be adaptable for interstellar missions, but for interplanetary work it seemed worth a look.
But the numbers were intractable. The magnetic scoop Andrews hoped to deploy created more drag than the ion engines produced thrust. The two researchers quickly found that the scoop’s best function was as a magnetic sail, and their work on the idea appeared in the literature in the early 1990s. In his 1999 book Entering Space, Zubrin recalls that the time was right for the magsail given that Paul Chu (University of Houston) had just invented the first high-temperature superconductors, which a magsail could theoretically use to create the magnetic field that would allow it to ride on the solar wind. Practical high-temperature superconducting wire born out of this work might one day allow magsails to achieve higher thrust-to-weight ratios than solar sails.
Magsails have clear propulsion implications, but Zubrin states the obvious about their most effective uses:
…the most interesting and important thing about the magsail is not what it can do to speed up a spacecraft — what’s important is its capability for slowing one down. The magsail is the ideal interstellar mission brake! No matter how fast a spaceship is going, all it has to do to stop is deploy and turn on a magsail, and the drag generated against the interstellar plasma will do the rest. Just as in the case of a parachute deployed by a drag racer, the faster the ship is going, the more ‘wind’ is felt, and the better it works.Which takes us to the idea of using in-situ resources to tackle the deceleration problem. If your goal is to launch a starship that can decelerate in the destination system to explore it, the magsail lets you do the job without carrying the deceleration fuel aboard the vehicle. Play around with the numbers long enough and you’ll see what a huge boost this would be, for otherwise you’re carrying all the fuel needed to slow down a starship (moving, perhaps, at .10 c!), and that means you’ve got to get all of that fuel up to cruise in the first place. The idea of creating drag against the interstellar medium and a destination stellar wind thus has a powerful appeal.
Rise of the Superconductor
When Bussard studied how his ramjet could operate in a region of interstellar space where the density of hydrogen was roughly 1 hydrogen atom per cubic centimeter, he saw that he would need a collecting area of 10,000 square kilometers. This is so vast that even if it were made of 0.1-centimeter mylar, a physical scoop would weigh something on the order of 250,000 tons. But a much smaller collector generating a magnetic field seems practical given the advances in superconducting alluded to above, with a loop of superconducting wire deployed from the spacecraft, the current applied to it cycling continuously to generate the magnetic field. Here’s how Zubrin and Andrews described it in a paper based on their presentation at the 1990 Vision-21 symposium at NASA’s Lewis Research Center (now Glenn Research Center):
The magnetic sail, or Magsail, is a device which can be used to accelerate or decelerate a spacecraft by using a magnetic field to accelerate/deflect the plasma naturally found in the solar wind and interstellar medium. Its principle of operation is as follows: A loop of superconducting cable hundreds of kilometers in diameter is stored on a drum attached to a payload spacecraft. When the time comes for operation the cable is played out into space and a current is initiated in the loop. This current once initiated, will be maintained indefinitely in the superconductor without further power. The magnetic field created by the current will impart a hoop stress to the loop aiding the deployment and eventually forcing it to a rigid circular shape.
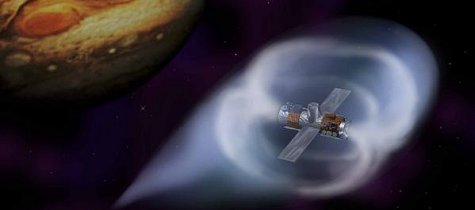
Image: A space probe surrounded by a magnetic sail. Early work
on these concepts has taken place at the University of Washington
under Robert Winglee, with reports available at NASA’s Institute
for Advanced Concepts site. Credit: NASA/University of Washington.
Thus the hybrid concept Andrews and Zubrin came up with in the Vision-21 work, extending ideas they had first presented in a 1988 paper: Use laser beaming technology to push a sail to interstellar cruise speeds, then deploy a magsail upon arrival to reduce deceleration time. The authors looked at the numbers and worked out 0.8 years for acceleration, 17.4 years of coasting at almost half the speed of light, and 18.8 years for deceleration. This gets you about 10 light years out in around 37 years, a mind-bending pace that uses a huge sail and some generous assumptions about laser power that we’ll look at tomorrow. For there are other ways to use lasers, even for deceleration, and other ways, too, to exploit the local interstellar medium.
Zubrin and Andrews’ paper from Vision-21 is “Use of Magnetic Sails for Advanced Exploration Missions,” in the proceedings of Vision-21: Space Travel for the Next Millennium” (NASA Conference Publication 10059. The citation for their 1988 work is given in yesterday’s Centauri Dreams post.
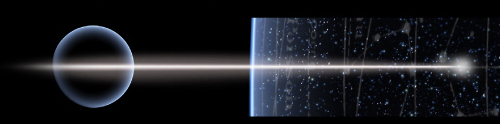
- March 19, 2012 at 11:48
- It may be possible to combine magsails and laser propulsion in another way. Use the magnetic field to store a plasma of partially ionized ions, for example singly ionized alkali earth elements, that have a strong absorption line. Illuminate the plasma with laser light at resonance, where the scattering cross section is extremely high. The laser would have to operate at variable frequency to compensate for doppler shift.
Such a sail should be able to tolerate extremely high laser intensity, since it comes pre-vaporized. If the laser is properly tuned, it could even remove thermal energy from the plasma by preferentially scattering off ions with velocities (in the frame of the vehicle) directed toward the laser. - March 19, 2012 at 13:44
- Won’t there be a back-emf on the loop
that requires power for deceleration?
If this were so if would make things worse
because of the inefficiency of generating electricity. - March 19, 2012 at 15:44
- Interstellar Bill, the braking isn’t via a magnetic motor effect at all. Instead the ISM plasma is being deflected by the magnetic field and it’s that change in momentum which is then transferred to the sail. There’s no electrical currents or EMFs involved in the plasma flow itself as such. The spatial density of the charges is low enough that their electrical interactions can be ignored, and there’s no net charge in the ISM. There is some charge separation in the magnetopause that forms in the initial shock front, which only enhances the coupling between mag-sail and ISM.
- March 19, 2012 at 19:00
- Then it would be nice if the destination star had a strong stellar wind, with a dense plasma for the loop to grab. High-mass stars look good.
As for superconducting loops, for sure unshielded, do we know their vulnerability to the considerable radiation hazards of interstellar flight?
For example, their superconductivity may get poisoned by radiation-generated defects, their mass eroded by collision-induced spallation.
The longer and faster the trip the worse are such problems. Perhaps the loop could be continuously repaired by crawling nanobots, or perhaps it could be kept in a shielded compartment to be deployed at:
ShowTime,
the Big SlowDown,
the Mandatory Halt,
the Blessed Trip-Arrival.
I can see a considerable potential here for the Galactic Religion to have tremendous ceremonies of euphoric triumph and elated congratulation to greet starship arrivals.
Imagine being finally slowed down and seeing a Sun again,
after all that time HURTLING THE COLD DANGEROUS DARK.
Now that’s a Salvation Experience. - March 19, 2012 at 21:36
- The incredibly low density of the interstellar medium and the very short distances (compared to interstellar distances) that stellar wind will be available are formidable problems here. Another one is that the same wind you want to brake against also tends to blow away the field you’d like to brake with. There is going to be a maximum current density in that wire, and with that comes a maximum extent of magnetosphere around it, which will diminish with increasing speed.
I have heard very little quantitative arguments, mostly hand waving. Dana and Zubrin, JBIS 1990, have an interesting quantitative treatment (path-2.narod.ru/design/base_e/msit.pdf), and they claim an acceleration in the solar wind of 0.01 m/s^2, and interstellar breaking of a factor of e in five years. Not great, but perhaps just enough to be useful? Neither of these take payload into account, I think.
The “sweeping away” of magnetic field lines, which is well known from the magnetospheres of the planets, has not been considered as far as I can tell. It should make a huge dent in the already weak performance, I would think. Has anyone looked into this? - March 19, 2012 at 21:48
- Many scientists have picked up the “brain bug” that says that starships must carry all the fuel and propellent they use to speed up and slow down- but the whole concept of magnetic sails shows that this utterly untrue. I am confident that magnetic sails will be a liberating technology, both in our solar system and beyond. Magnetic sails can be used to shield orbiting space colonies from cosmic rays, gather hydrogen from the solar wind, propel spacecraft, and brake interstellar craft without expelling propellent- that is a lot of uses for one technology!!
- March 20, 2012 at 1:56
- Could it be possible to use a laser of the most useful wavelength in a cone shape configuration ‘the base edge’ to move gas ahead of the craft into the magsails path and ionise it at the same time, a sort of long range gatherer. It would collect a much larger amount of interstellar and alien solar wind gases.
- March 21, 2012 at 0:18
- There are a few big problem with making a big sail.
First the energy is a function of the SIZE of the field as well as its strength. thus a big sale takes a lot of energy to construct (inflate? energize?) . Second the forces on the wire loop are not trivial, with the loop tending to collapse as the field builds. a really big loop is hard to keep open since it need to be kilometers in diameter.
I thought of spinning the loop to supply an inertial ( “centrifugal”) force to keep it open. - March 21, 2012 at 18:31
- @jkittle: I believe the loop would tend to expand from the field it generates, thus be stabilized. It is even possible that its tensile strength puts a more stringent limit on the field strength per mass than the current density. Spinning the loop is a good idea, but will not be necessary.
Christopher Phoenix: Indeed, it would be great if magnetic sails could do all these wonderful things, but unfortunately when you do the numbers with realistic assumptions they come up short on most counts. Sometimes a “brain bug” is simply a realization that fantasy won’t move your ship. - March 21, 2012 at 23:24
- actually the lopp will tend to crunch closed, this is one of the limitations on practical construction of high magnetic field coils used in NMR ( nuclear magnetic Resonance) or in particle accelerators like CERN. the force on the wires become extreme
- March 22, 2012 at 20:09
- When I was a teenager, the Bussard Ramscoop had a lot of appeal for serious Interstellar man rated travel. The sufficiency of Alpha-Hydrogen, the architecture for advance fusion powered propulsion…. about several dozen ‘sobering’ assessments that put the idea on the shelf until science ‘catches up’ or supercedes this design?
But what seems to be contradictory in speculating about advanced/exotic deep space flight is that half the conditions are ‘listed’ as improbable or infeasible to accelerate a spaceship… and the same list will erode or ‘fry’ a spaceship!
I understand the principle of diminishing return, but there should come a point where a ‘technology’ field demonstration can answer these concerns.
I’m tired of the ‘about 300 years to never’ comments in solving these problems… its almost like arguing with Creationists about ‘great’ certainty’ without ‘confirming’ evidence. - March 23, 2012 at 15:33
- @jkittle: Actually, the force is outwards. Read the sec
A Force Field for Astronauts?
Researchers are reviving an old but wild idea to protect astronauts from space radiation.
June 24, 2005: Opposite charges attract. Like charges repel. It's the first lesson of electromagnetism and, someday, it could save the lives of astronauts.
NASA's Vision for Space Exploration calls for a return to the Moon as preparation for even longer journeys to Mars and beyond. But there's a potential showstopper: radiation.
Space beyond low-Earth orbit is awash with intense radiation from the Sun and from deep galactic sources such as supernovas. Astronauts en route to the Moon and Mars are going to be exposed to this radiation, increasing their risk of getting cancer and other maladies. Finding a good shield is important.
Right: Supernovas produce dangerous radiation. [More]The most common way to deal with radiation is simply to physically block it, as the thick concrete around a nuclear reactor does. But making spaceships from concrete is not an option. (Interestingly, it might be possible to build a moonbase from a concrete mixture of moondust and water, if water can be found on the Moon, but that's another story.) NASA scientists are investigating many radiation-blocking materials such as aluminum, advanced plastics and liquid hydrogen. Each has its own advantages and disadvantages.
Most of the dangerous radiation in space consists of electrically charged particles: high-speed electrons and protons from the Sun, and massive, positively charged atomic nuclei from distant supernovas.
Like charges repel. So why not protect astronauts by surrounding them with a powerful electric field that has the same charge as the incoming radiation, thus deflecting the radiation away?
Many experts are skeptical that electric fields can be made to protect astronauts. But Charles Buhler and John Lane, both scientists with ASRC Aerospace Corporation at NASA's Kennedy Space Center, believe it can be done. They've received support from the NASA Institute for Advanced Concepts, whose job is to fund studies of far-out ideas, to investigate the possibility of electric shields for lunar bases.
Above: Artist’s concept of an electrostatic radiation shield,consisting of positively charged inner spheres and negativelycharged outer spheres. The screen net is connected to ground.Image courtesy ASRC Aerospace.
Buhler and Lane's approach is different. In their concept, a lunar base would have a half dozen or so inflatable, conductive spheres about 5 meters across mounted above the base. The spheres would then be charged up to a very high static-electrical potential: 100 megavolts or more. This voltage is very large but because there would be very little current flowing (the charge would sit statically on the spheres), not much power would be needed to maintain the charge.
The spheres would be made of a thin, strong fabric (such as Vectran, which was used for the landing balloons that cushioned the impact for the Mars Exploration Rovers) and coated with a very thin layer of a conductor such as gold. The fabric spheres could be folded up for transport and then inflated by simply loading them with an electric charge; the like charges of the electrons in the gold layer repel each other and force the sphere to expand outward.
Right: How the voltage would vary above a lunar base for the sphere configuration shown above. You can learn more about this and other configurations in the report Analysis of a Lunar Base Electrostatic Radiation Shield Concept.Placing the spheres far overhead would reduce the danger of astronauts touching them. By carefully choosing the arrangement of the spheres, scientists can maximize their effectiveness at repelling radiation while minimizing their impact on astronauts and equipment at the ground. In some designs, in fact, the net electric field at ground level is zero, thus alleviating any potential health risks from these strong electric fields.
Buhler and Lane are still searching for the best arrangement: Part of the challenge is that radiation comes as both positively and negatively charged particles. The spheres must be arranged so that the electric field is, say, negative far above the base (to repel negative particles) and positive closer to the ground (to repel the positive particles). "We've already simulated three geometries that might work," says Buhler.
Portable designs might even be mounted onto "moon buggy" lunar rovers to offer protection for astronauts as they explore the surface, Buhler imagines.
Right: One scenario for how an electrostatic radiation shield could be deployed for mobile lunar exploration vehicles. Inverted green cones denote regions of partial radiation protection. Image courtesy ASRC Aerospace.It sounds wonderful, but there are many scientific and engineering problems yet to be solved. For example, skeptics note that an electrostatic shield on the Moon is susceptible to being short circuited by floating moondust, which is itself charged by solar ultraviolet radiation. Solar wind blowing across the shield can cause problems, too. Electrons and protons in the wind could become trapped by the maze of forces that make up the shield, leading to strong and unintended electrical currents right above the heads of the astronauts.
The research is still preliminary, Buhler stresses. Moondust, solar wind and other problems are still being investigated. It may be that a different kind of shield would work better, for instance, a superconducting magnetic field. These wild ideas have yet to sort themselves out.
But, who knows, perhaps one day astronauts on the Moon and Mars will work safely, protected by a simple principle of electromagnetism even a child can understand.
Author: Patrick L. Barry | Editor: Dr. Tony Phillips | Credit: Science@NASA
Force Fields
Radiation shields composed of matter are quite massive, and Every Gram Counts. Researchers have been looking into using magnetic and electrostatic fields to protect against particle radiation, since such fields have no mass. Unfortunately the generators of such fields do have mass. And the field strength will have to be strong enough that the word "superconductor" will soon be mentioned. In addition, such powerful fields might be health hazards to the astronauts. It is worthless if the field simultaneously protects the astronaut from particle radiation, but also instantly kills them by being strong enough to straighten out all their DNA molecules.
Electromagnetic particle shield from Soviet magazine (1963)
Temperature Superconducting (HTS) Magnets
The work performed showed that single layer expandable coils with diameters of 4 to 8 m and lengths of 15 to 20 m, arranged in a 6-around-1 configuration constitute the best solution among all concepts analyzed. The single compensator coil closely surrounds the habitat serving as a habitat thermal radiation shield for the outer coils and compensates for outer coil fringe fields trying to enter the habitat.
Electrostatic Sphere tree with positively charged inner spheres and negatively charge outer spheres. The yellow net is grounded.
From Analysis of a Lunar Base Electrostatic Radiation Shield Concept (2005)
Electric field potential along z-axis for negative-positive sphere tree.Electrostatic sphere tree with only postively charged spheres
From Analysis of a Lunar Base Electrostatic Radiation Shield Concept (2005)
Electric field potential along z-axis for positive sphere tree.Plasma bubble shield concept by John Slough of the University of Washington
Ionized hydrogen is confined by a superconducting net to provide radiation shielding. Bubble is 100 meters in diameter, is as effective at stopping radiation as ten centimeters of aluminum, but has much less mass.
Magnetic Shield
Shielding system is about nine tons, which is much less that material anti-radiation shields but still a disappointingly large chunk to remove from the payload capacity. To stop cosmic rays within a few meters the field strength will have to be about 20 teslas (600,000 times that of Terra).
Pros: much lighter than mateiral shield
Cons: offers no protection along the axis (which is why the living quarters are donut shaped). 20 teslas might be harmful to human beings.
From Shielding Space Travelers by Eugene N. Parker, Scientific American March 2006
Magnetic Shield
In this design, a second inner magnet partially cancels out the 20 tesla field inside the living quarters.
Pros: the magnetic field inside the living quarters is lower
Cons: the magnetic field inside the living quuarters is still much higher than on Terra. The system has more mass and more points of failure.
From Shielding Space Travelers by Eugene N. Parker, Scientific American March 2006
This concept uses the Mini-magnetospheric plasma sail propulsion system (M2P2) as an anti-radiation shield method.
From An exploration of the effectiveness of artificial mini-magnetospheres as a potential Solar Storm shelter for long term human space missions (2014)
The deflection of a high energy ion (green) by the electric field (Er) (red) created by the low energy plasma captured and retained by the magnetic field from the spacecraft. Augmenting the natural density, by releasing readily ionised gas from the craft, can enable protection of >> 100MeV/amu ions.
http://www.mnn.com/
Can these physicists create an engine propelled by antimatter?
The warp drive in the USS Enterprise is fueled by a matter-antimatter chamber. These physicists are trying to create amore realistic version. (Photo: Paramount)Physicists Gerald Jackson and Steven Howe want to develop an engine that will propel spacecraft at 40 percent the speed of light, and they’re relying on Kickstarter to make it happen.
The duo behind Chicago's HBar Technologies (the slogan of which is “Making Antimatter Matter") plan to set a Kickstarter goal of $200,000, which seems like a reasonable price for bringing science fiction to reality. However, that $200,000 only covers the funding of proof of concept — a device that would measure the output of the antimatter engine. Building the real antimatter-fueled probe would take an estimated $100 million. However, if they prove their engine could be a reality, Jackson and Howe predict that heavy hitters like NASA would foot the rest of the bill.
Antimatter propulsion will sound familiar to any "Star Trek" fans out there. In fact, the fictional USS Enterprise’s warp drive was fueled by the warp core, a matter-antimatter chamber. Hypothetically, Jackson and Howe's spacecraft is a more realistic version of this, but only if they can harness the power of antimatter.
Jackson and Howe aren’t operating entirely on speculation. They are building on years and years of research by others, and a report from the 2003 Particle Accelerator Conference (pdf) reveals that they’ve refined the plans for a space probe. In theory, it could work and change space exploration forever. Scientists have been researching antimatter propulsion for years, but Jackson and Howe are the first to crowdfund the endeavor.
Popular Science explains how Jackson and Howe’s antimatter-fueled probe would function: “The design would use antimatter to induce a fission reaction. During this reaction, uranium would split into two 'daughter' byproducts. One of the daughters flies forward, striking into a sail and propelling it forward, similar to how the wind pushes a sailboat. The other daughter particle would shoot out the back of the spacecraft, creating another source of thrust.”
Aside from the enormous cost of Jackson and Howe’s project, there are logistical and safety concerns that could prevent their success. Antimatter, which is just like regular matter except is has an opposite charge, is extremely volatile. It destroys any particle of matter it comes into contact with, creating the powerful release of energy that Jackson and Howe want to use to propel a craft into deep space. The release of energy is also what makes the stuff so dangerous. Jackson and Howe’s report states that they’d need 17 grams of it to make their engine work. Also, antimatter is hard to contain, since it destroys every particle of matter it touches. In short, you have to contain it very carefully.
Aside from those challenges, there's the problem that antimatter is hard to come by; only supercolliders like CERN and Jackson’s former employer Fermilab are capable of creating it. It’s also expensive to make. Symmetry Magazine, a publication dedicated to particle physics, explains, “The problem lies in the efficiency and cost of antimatter production and storage. Making 1 gram of antimatter would require approximately 25 million billion kilowatt-hours of energy and cost over a million billion dollars.”
A $200,000 Kickstarter goal (and even $100 million in additional funding) is barely a drop in that expensive bucket.
However, if antimatter production becomes more efficient and therefore more affordable, dreams for an antimatter propulsion engine could become a reality. If being a part of this experiment appeals to you, watch out for the Kickstarter campaign when it launches later this month.NASA Has Designed a Warp Ship Inspired by ‘Star Trek’s Enterprise
1 day ago
When does science-fiction become science fact? Throughout various mediums over the last few centuries, we’ve seen early versions of concepts that would eventually become a reality. Sometimes these portrayals are pretty far off base (still waiting on those flying cars), while other times they feel downright prescient. But in the case of Star Trek and one particular engineer at NASA, science-fiction actually informed science fact, with NASA engineer and physicist Harold White now actively working on a space ship that would allow travel faster than the speed of light—or, for the Star Trek inclined, warp speed.
White announced this idea a few years ago, with the concept seeking to allow travel faster than the speed of light by literally expanding space-time behind the object and contracting space-time in front of it. In reality, the object doesn’t “go fast,” but instead takes advantage of Albert Einstein’s theory of relativity to move between space-time.
If your head has yet to explode, sit tight—in concert with White, designer Mark Rademaker has now created a CGI design concept of the ship that would operate using this theory, which they have aptly named the IXS Enterprise. Per Rademaker in an interview with the Washington Post, the idea behind the concept art serves two purposes: to visualize their idea, and to inspire burgeoning young scientists:
“We wanted to have a decent image of a theory conforming Warp ship to motivate young people to pursue a STEM career,” Rademaker said in an e-mail interview. “It does have some Sci-Fi features that might never transfer to a possible final design, unless we really want to.”
So how close is this IXS Enterprise to becoming a reality? Well, the first step is proving that the math is realistically sound, which is what White is working on now. Still, this is kind of great, and it’s neat to see science-fiction being used to help actualize an idea that could radically change humanity’s place in the universe as we know it. See kids, science is cool!
Flip through Rademaker’s concept images below, followed by a video in which White explains the science behind this warp ship in much greater detail.
Images used with permission by Mark Rademaker
No comments:
Post a Comment