In a new experimental system, the concept of "light cones" doesn't apply.
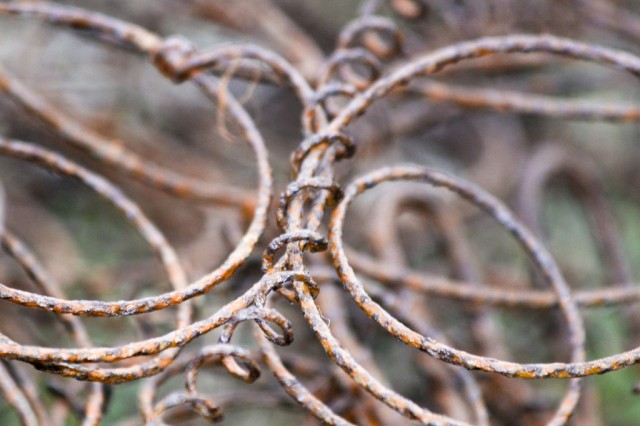
It’s tempting to think that quasiparticles are not particles at all. Quasiparticles are “objects” that emerge within a complex system, such as a solid object. The collective behavior of the particles in the solid can create the impression of a new particle. The impression—or quasiparticle—moves through the solid as if it were a real particle moving through empty space, and it behaves according to the same rules.
Nevertheless, within their system, quasiparticles can have real effects on their environment. Most recently, scientists were able to track the propagation of quasiparticles called magnons through a collection of atoms. Now, scientists have been able to watch as that propagation changed the behavior of these atoms. And in the process, the quasiparticles reached speeds where a conventional model, which we use to understand time, breaks down.
To make these observations, the researchers lined up seven ions and targeted the fourth ion, exactly in the middle of the line, with a laser. The laser changes the ion’s quantum spin direction.
Changing the spin of the fourth (middle) ion sends out quasiparticles in both directions, much in the same way that a pebble, dropped into a pond, sends out a ripple in all directions.
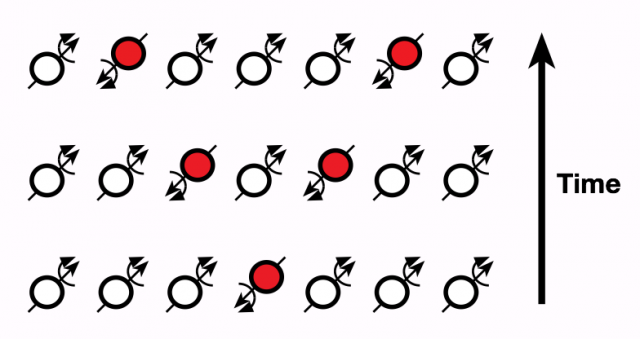
John Timmer
As the two magnons moved away from the middle of the line, entanglement moved with them. That is, as the magnon moving to the right passed over ion 5, and as the one moving to the left passed over ion 3, ions 3 and 5 became entangled with each other.
The scientists were able to measure how the entanglement changed with time as the two magnons propagated away from each other. Their results agreed very closely with prediction—pairs of ions were briefly measured to be entangled as the pair of magnons moved over them, and then ceased to be entangled once the magnon was gone.
The experiment also had a second layer. The scientists were able to “tune” the range of interactions between the ions in the system. In other words, they could adjust how far one ion’s influence on its neighbors reaches. In the first part of the experiment, each ion’s spin essentially only influenced its immediate neighbors’. In the second, the researchers were able to adjust it so that the ions’ spin can jump over adjacent ions, changing the spins of more distant ones.
The resulting collective behavior of the ions still produced quasiparticles, but quasiparticles of a different sort, moving at a different speed. As they tuned the system to three different interaction ranges, the quasiparticles became faster and faster, ultimately approaching infinite speed.
Actual infinite speed is not possible, even in a quantum system, due to a speed limit called the Lieb-Robinson bound. The actual top speed of a quasiparticle may vary depending on the system it inhabits, but it is always finite. However, according to the researchers, the Lieb-Robinson bounds are “trivial” in certain circumstances, such as in their tuned system, meaning that there’s essentially no restriction on the speeds of the quasiparticles under certain circumstances.
The unbounded speed also breaks down conventional notions of time. A relativistic model called the light-cone is often used to understand time. Light-cones are graphs of the furthest light beams that can reach an object given a certain time. Nothing can travel faster than the speed of light, so only objects in the “past” part of an object’s light-cone can possibly transfer information to that object.
This model holds in the first part of the experiment, but once the researchers had tuned the interaction ranges of the ions, they found that the speeds of the quasiparticles were such that they could no longer be described in terms of light-cones.
The experiment is significant not only for its findings, which agree closely with prediction (and are the first time entanglement due to quasiparticles has been observed), but also because it lays the groundwork for many future avenues of study.
Experiments like this one, involving many-body systems, are crucial to our understanding of a wide range of quantum phenomena.
Nature, 2014. DOI: 10.1038/nature13461 (About DOIs).
No comments:
Post a Comment