From Wikipedia, the free encyclopedia

Graphene is an atomic-scale honeycomb lattice made of carbon atoms.
At the time of its isolation, many researchers studying carbon nanotubes were already well familiar with the composition, structure and properties of graphene which had been calculated decades earlier. The combination of familiarity, extraordinary properties and surprising ease of isolation enabled an explosion in graphene research. The Nobel Prize in Physics for 2010 was awarded to Andre Geim and Konstantin Novoselov at the University of Manchester "for groundbreaking experiments regarding the two-dimensional material graphene".[1]
Contents
- 1 Description
- 2 Occurrence and production
- 3 Atomic structure
- 4 Properties
- 5 Forms
- 6 Potential applications
- 6.1 Electronics
- 6.2 Redox
- 6.3 Transparent conducting electrodes
- 6.4 Ethanol distillation
- 6.5 Desalination
- 6.6 Solar cells
- 6.7 Single-molecule gas detection
- 6.8 Quantum dots
- 6.9 Frequency multiplier
- 6.10 Optical modulators
- 6.11 Additives in coolants
- 6.12 Reference Material
- 6.13 Thermal management materials
- 6.14 Energy storage
- 6.15 Engineered piezoelectricity
- 6.16 Biodevices
- 7 Pseudo-relativistic theory
- 8 History and experimental discovery
- 9 See also
- 10 References
- 11 External links
Description
Graphene is an allotrope of carbon whose structure is a single planar sheet of sp2-bonded carbon atoms, that are densely packed in a honeycomb crystal lattice.[2] The term graphene was coined as a combination of graphite and the suffix -ene by Hanns-Peter Boehm,[3] who described single-layer carbon foils in 1962.[4] Graphene is most easily visualized as an atomic-scale chicken wire made of carbon atoms and their bonds.The carbon-carbon bond length in graphene is about 0.142 nanometers.[5] Graphene sheets stack to form graphite with an interplanar spacing of 0.335 nm. Graphene is the basic structural element of some carbon allotropes including graphite, charcoal, carbon nanotubes and fullerenes. It can also be considered as an indefinitely large aromatic molecule, the limiting case of the family of flat polycyclic aromatic hydrocarbons.
The IUPAC compendium of technology states: "previously, descriptions such as graphite layers, carbon layers, or carbon sheets have been used for the term graphene... it is incorrect to use for a single layer a term which includes the term graphite, which would imply a three-dimensional structure. The term graphene should be used only when the reactions, structural relations or other properties of individual layers are discussed."[6] In this regard, graphene has been referred to as an infinite alternant (only six-member carbon ring) polycyclic aromatic hydrocarbon (PAH). The largest known isolated molecule of this type consists of 222 atoms and is 10 benzene rings across.[7] It has proven difficult to synthesize even slightly bigger molecules, and they still remain "a dream of many organic and polymer chemists".[8]
Furthermore, ab initio calculations show that a graphene sheet is thermodynamically unstable with respect to other fullerene structures if its size is less than about 20 nm (“graphene is the least stable structure until about 6000 atoms”) and becomes the most stable one (as within graphite) only for sizes larger than 24,000 carbon atoms.[9] The flat graphene sheet is also known to be unstable with respect to scrolling i.e. curling up, which is its lower-energy state.[10]
A definition of "isolated or free-standing graphene" has also recently been proposed: "graphene is a single atomic plane of graphite, which – and this is essential – is sufficiently isolated from its environment to be considered free-standing."[11] This definition is narrower than the definitions given above and refers to cleaved, transferred and suspended graphene monolayers.[citation needed]
Other forms of graphene, such as graphene grown on various metals, can also become free-standing if, for example, suspended or transferred to silicon dioxide (SiO2). A new example of isolated graphene is graphene on silicon carbide (SiC) after its passivation with hydrogen.[12]
Potential applications
Several potential applications for graphene are under development, and many more have been proposed. These include lightweight, thin, flexible, yet durable display screens, electric circuits, and solar cells, as well as various medical, chemical and industrial processes enhanced or enabled by the use of new graphene materials.[156]In 2013, graphene researchers led by Prof. Jari Kinaret from Sweden's Chalmers University of Technology, secured a €1 billion grant from the European Union to be used for further research into development of potential applications of graphene.[157] In 2013 was formed Graphene Flagship consortium including Chalmers University of Technology and next seven European universities and research centers, and Finland company Nokia.[158][159] Nokia has also been working on graphene technology for several years.[160]
Electronics
Integrated circuits
Graphene has the ideal properties to be an excellent component of integrated circuits. Graphene has a high carrier mobility, as well as low noise, allowing it to be used as the channel in a field-effect transistor. The issue is that single sheets of graphene are hard to produce, and even harder to make on top of an appropriate substrate. Researchers are looking into methods of transferring single graphene sheets from their source of origin (mechanical exfoliation on SiO2 / Si or thermal graphitization of a SiC surface) onto a target substrate of interest.[161] In 2008, the smallest transistor so far, one atom thick, 10 atoms wide was made of graphene.[162] IBM announced in December 2008 that they fabricated and characterized graphene transistors operating at GHz frequencies.[163] In May 2009, an n-type transistor was announced meaning that both n and p-type transistors have now been created with graphene.[164][165] A functional graphene integrated circuit was also demonstrated – a complementary inverter consisting of one p- and one n-type graphene transistor.[166] However, this inverter also suffered from a very low voltage gain.According to a January 2010 report,[167] graphene was epitaxially grown on SiC in a quantity and with quality suitable for mass production of integrated circuits. At high temperatures, the Quantum Hall effect could be measured in these samples. See also the 2010 work by IBM in the transistor section above in which 'processors' of 100 GHz transistors on 2-inch (51 mm) graphene sheets were made.[168]
In June 2011, IBM researchers announced that they had succeeded in creating the first graphene-based integrated circuit, a broadband radio mixer.[169] The circuit handled frequencies up to 10 GHz, and its performance was unaffected by temperatures up to 127 degrees Celsius.
In June 2013 an 8 transistor 1.28 GHz ring oscillator circuit was described.[170]
Transistors
Graphene exhibits a pronounced response to perpendicular external electric fields, potentially forming field-effect transistors. A 2004 paper documented FETs with an on-off ratio of ~30 at room temperature.[citation needed] A 2006 paper announced an all-graphene planar FET with side gates.[171] Their devices showed changes of 2% at cryogenic temperatures. The first top-gated FET (on–off ratio of <2) was demonstrated in 2007.[172] Graphene nanoribbons may prove generally capable of replacing silicon as a semiconductor.[173]A 2008 paper demonstrated a new switching effect based on a reversible chemical modification of the graphene layer that gives an on–off ratio of greater than six orders of magnitude. These reversible switches could potentially be applied to nonvolatile memories.[174]
In 2009, researchers demonstrated four different types of logic gates, each composed of a single graphene transistor.[175]
Practical uses for these circuits are limited by the very small voltage gain they exhibit. Typically, the amplitude of the output signal is about 40 times less than that of the input signal. Moreover, none of these circuits operated at frequencies higher than 25 kHz.
In the same year, tight-binding numerical simulations[176] demonstrated that the band-gap induced in graphene bilayer field effect transistors is not sufficiently large for high-performance transistors for digital applications, but can be sufficient for ultra-low voltage applications, when exploiting a tunnel-FET architecture.[177]
In February 2010, researchers announced transistors with an on/off rate of 100 gigahertz, far exceeding the rates of previous attempts, and exceeding the speed of silicon transistors with an equal gate length. The 240 nm devices were made with conventional silicon-manufacturing equipment.[178][179][180]
In November 2011, researchers used ink-jet printing (additive manufacturing) as a method for fabricating graphene devices.[181]
In 2013, researchers demonstrated graphene's high mobility in a detector that allows broad band frequency selectivity ranging from the THz to IR region (0.76-33THz)[182] A separate group created a terahertz-speed transistor with bistable characteristics, which means that the device can spontaneously switch between two electronic states. The device consists of two layers of graphene separated by an insulating layer of boron nitride just a few atomic layers thick. Electrons move through this barrier by quantum tunneling. These new transistors exhibit “negative differential conductance,” whereby the same electrical current flows at two different applied voltages.[183][184]
Graphene does not have an energy band-gap, which presents a hurdle for its applications in digital logic gates. The efforts to induce a band-gap in graphene via quantum confinement or surface functionalization have not resulted in a breakthrough. A team of researchers at the University of California has shown that the negative differential resistance experimentally observed in graphene field-effect transistors of "conventional" design allows for construction of viable non-Boolean computational architectures with the gap-less graphene. The negative differential resistance - observed under certain biasing schemes - is an intrinsic property of graphene resulting from its symmetric band structure. The obtained results present a conceptual change in graphene research and indicate an alternative route for graphene's applications in information processing.[185]
Also in 2013, researchers reported the creation of transistors printed on flexible plastic that operate at 25-gigahertz , sufficient for communications circuits and can be fabricated at scale. To make the transistors, the researchers first fabricate all the non-graphene-containing structures—the electrodes and gates—on sheets of plastic. Separately, they grow large sheets of graphene on metal, then peel it off and transfer it to the plastic. Finally, they top the sheet with a waterproof layer. The devices still work after being soaked in water, and they’re flexible enough to be folded up.[186]
Redox
Graphene oxide can be reversibly reduced and oxidized using electrical stimulus. Controlled reduction and oxidation in two-terminal devices containing multilayer graphene oxide films are shown to result in switching between partially reduced graphene oxide and graphene, a process that modifies the electronic and optical properties. Oxidation and reduction are also shown to be related to resistive switching.[187][188]Transparent conducting electrodes
Graphene's high electrical conductivity and high optical transparency make it a candidate for transparent conducting electrodes, required for such applications as touchscreens, liquid crystal displays, organic photovoltaic cells, and organic light-emitting diodes. In particular, graphene's mechanical strength and flexibility are advantageous compared to indium tin oxide, which is brittle, and graphene films may be deposited from solution over large areas.[189][190]Large-area, continuous, transparent, and highly conducting few-layered graphene films were produced by chemical vapor deposition and used as anodes for application in photovoltaic devices. A power conversion efficiency (PCE) up to 1.71% was demonstrated, which is 55.2% of the PCE of a control device based on indium-tin-oxide.[191]
Organic light-emitting diodes (OLEDs) with graphene anodes have also been demonstrated.[192] The electronic and optical performance of devices based on graphene are shown to be similar to devices made with indium-tin-oxide.
An all carbon-based device called a light-emitting electrochemical cell (LEC) was demonstrated with chemically derived graphene as the cathode and the conductive polymer PEDOT as the anode by Matyba et al.[193] Unlike its predecessors, this device contains no metal, but only carbon-based electrodes. The use of graphene as the anode in LECs was also verified in the same publication.
Ethanol distillation
Graphene oxide membranes allow water vapor to pass through, but have been shown to be impermeable to all other liquids and gases including helium .[143] This phenomenon has been used for further distilling of vodka to higher alcohol concentrations, in a room-temperature laboratory, without the application of heat or vacuum normally used in traditional distillation methods.[194] Further development and commercialization of such membranes could revolutionize the economics of biofuel production and the alcoholic beverage industry.Desalination
Research suggests that graphene filters could outperform other techniques of desalination by a significant margin.[195]Solar cells
Graphene has a unique combination of high electrical conductivity and optical transparency, which make it a good candidate for use in solar cells. A single sheet of graphene is a zero-bandgap semiconductor whose charge carriers are delocalized over large areas, implying that carrier scattering does not occur. Because this material only absorbs 2.3% of visible light, it is a candidate for applications as a transparent conductor.[196] Graphene can be assembled into a film electrode with low roughness. However, in practice, graphene films produced via solution processing contain lattice defects and graon boundaries that act as recombination centers and decrease the electrical conductivity of the material. Thus, these films must be made thicker than one atomic layer in order to obtain sheet resistances that are sensible. This added resistance can be combatted by incorporating conductive filler materials, such as a silica matrix. Reduced Graphene film's electrical conductivity can also be improved by attaching large aromatic molecules such as pyrene-1-sulfonic acid sodium salt (PyS) and the disodium salt of 3,4,9,10-perylenetetracarboxylic diimide bisbenzenesulfonic acid (PDI). These large aromatic molecules, under high temperatures, facilitate better π-conjugation of the graphene basal plane. Graphene films have high transparency in the visible and near-infrared regions, and are also highly chemically and thermally stable.[196]In order for graphene to be put to use in solar cells commercially, large-scale production of the material would need to be achieved. However, the peeling of pyrolytic graphene does not seem to be a simple process to scale up. An alternative method with potential for scalable production of graphene that has been suggested is the thermal decomposition of silicon carbide.[196]
Other than graphene's use as a transparent conducting oxide (TCO), it has also exhibited high charge mobilities that lead one to conclude that it could be put to use as a charge collector and transporter in PVs. The use of graphene in OPVs as a photoactive material requires the bandgap to be tuned to within the range of 1.4-1.9eV. In 2010, Yong & Tour reported single cell efficiencies of nano structured graphene-based PVs of over 12%.[196] According to P. Mukhopadhyay and R. K. Gupta the future of graphene in OPVs could be "devices in which semiconducting graphene is used as the photoactive material and metallic graphene is used as the conductive electrodes".[197]
The USC Viterbi School of Engineering lab reported the large scale production of highly transparent graphene films by chemical vapor deposition in 2008. In this process, researchers create ultra-thin graphene sheets by first depositing carbon atoms in the form of graphene films on a nickel plate from methane gas. Then they lay down a protective layer of thermoplastic over the graphene layer and dissolve the nickel underneath in an acid bath. In the final step they attach the plastic-protected graphene to a very flexible polymer sheet, which can then be incorporated into an OPV cell (graphene photovoltaics). Graphene/polymer sheets have been produced that range in size up to 150 square centimeters and can be used to create dense arrays of flexible OPV cells. It may eventually be possible to run printing presses laying extensive areas covered with inexpensive solar cells, much like newspaper presses print newspapers (roll-to-roll).[198][199]
While silicon has long been the standard for commercial solar cells, new research from the Institute of Photonic Sciences (ICFO) in Spain has shown that graphene could prove far more efficient when it comes to transforming light into energy. The study found that unlike silicon, which generates only one current-driving electron for each photon it absorbs, graphene can produce multiple electrons. Solar cells made with graphene could offer 60% solar cell efficiency – double the widely-regarded maximum efficiency of silicon cells.[200]
Single-molecule gas detection
Theoretically graphene makes an excellent sensor due to its 2D structure. The fact that its entire volume is exposed to its surrounding makes it very efficient to detect adsorbed molecules. However, similar to carbon nanotubes, graphene has no dangling bonds on its surface. Gaseous molecules cannot be readily adsorbed onto graphene surface. So intrinsically graphene is insensitive.[201] The sensitivity of graphene chemical gas sensors can be dramatically enhanced by functionalizing graphene, for example, coating with a thin layer of certain polymers. The thin polymer layer acts like a concentrator that absorbs gaseous molecules. The molecule absorption introduces a local change in electrical resistance of graphene sensors. While this effect occurs in other materials, graphene is superior due to its high electrical conductivity (even when few carriers are present) and low noise, which makes this change in resistance detectable.[89]Quantum dots
Graphene quantum dots (GQDs) use graphene with all dimensions less than 100 nm. Their size and edge crystallography govern their electrical, magnetic, optical and chemical properties. GQDs can be produced via graphite nanotomy[202] or via bottom-up, solution-based routes (Diels-Alder, cyclotrimerization and/or cyclodehydrogenation reactions).[203] Several studies[162] have indicated that GQDs with controlled structure can be incorporated into applications in electronics, optoelectronics and electromagnetics. quantum confinement can be created by changing the width of GNRs at select points along the ribbon.[204]Frequency multiplier
In 2009, researchers built experimental graphene frequency multipliers that take an incoming signal of a certain frequency and outputs a signal at a multiple of that frequency.[205][206]Optical modulators
When the Fermi level of graphene is tuned, the optical absorption of graphene can be changed. In 2011, researchers at UC Berkeley reported the first graphene-based optical modulator. Operating at 1.2 GHz without any temperature controller, this modulator has a broad bandwidth (from 1.3 to 1.6 μm) and small footprint (~25 μm2).[207]Additives in coolants
Owing to high thermal conductivity of graphene, it could be used as additives in coolants. Preliminary research work has shown that 5.0 volume percentage graphene can enhance the thermal conductivity of a base fluid by 86%.[208] Another application due to enhanced thermal conductivity of graphene was found in polymerase chain reaction.[209]Reference Material
Graphene shows properties that enable it to be used as a reference material for characterizing electroconductive and transparent materials. One layer of graphene absorbs 2.3% of white light.[210] This property was used to define the conductivity of transparency that combines the sheet resistance and the transparency. This parameter was used to compare different materials without the use of two independent parameters.[211]Thermal management materials
In 2011, researchers in Georgia Institute of Technology reported that a three-dimensional vertically aligned functionalized multilayer graphene architecture can be an approach for graphene-based thermal interfacial materials (TIMs) with superior equivalent thermal conductivity and ultra-low interfacial thermal resistance between graphene and metal.[119]Energy storage
Ultracapacitors
Due to the extremely high surface area to mass ratio of graphene, one potential application is in the conductive plates of ultracapacitors. It is believed that graphene could be used to produce ultracapacitors with a greater energy storage density than is currently available.[212]Electrode for Li-ion batteries (microbatteries)
Stable Li-ion cycling has recently been demonstrated in bi and few layer graphene films grown on Nickel substrates,[213][214] while single layer graphene films have been demonstrated as a protective layer against corrosion in battery components such as the steel casing.[215] This opens up the possibilities for flexible electrodes for microscale Li-ion batteries where the anode acts as the active material as well as the current collector.[216]Engineered piezoelectricity
Density functional theory simulations predict that depositing certain adatoms on graphene can render it piezoelectrically responsive to an electric field applied in the vertical (i.e. out-of-plane) direction. This type of locally engineered piezoelectricity is similar in magnitude to that of bulk piezoelectric materials and make graphene a candidate tool for control and sensing in nanoscale devices.[217][218]Biodevices
Graphene's modifiable chemistry, large surface area, atomic thickness and molecularly gatable structure make antibody-functionalized graphene sheets excellent candidates for mammalian and microbial detection and diagnosis devices.[219]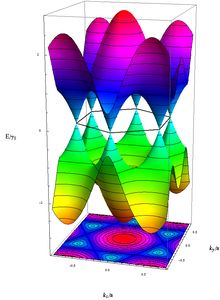
Energy of the electrons with wavenumber k in graphene, calculated in the Tight Binding-approximation. The unoccupied (occupied) states, colored in blue–red (yellow–green), touch each other without energy gap exactly at the above-mentioned six k-vectors.
No comments:
Post a Comment