https://www.newscientist.com/
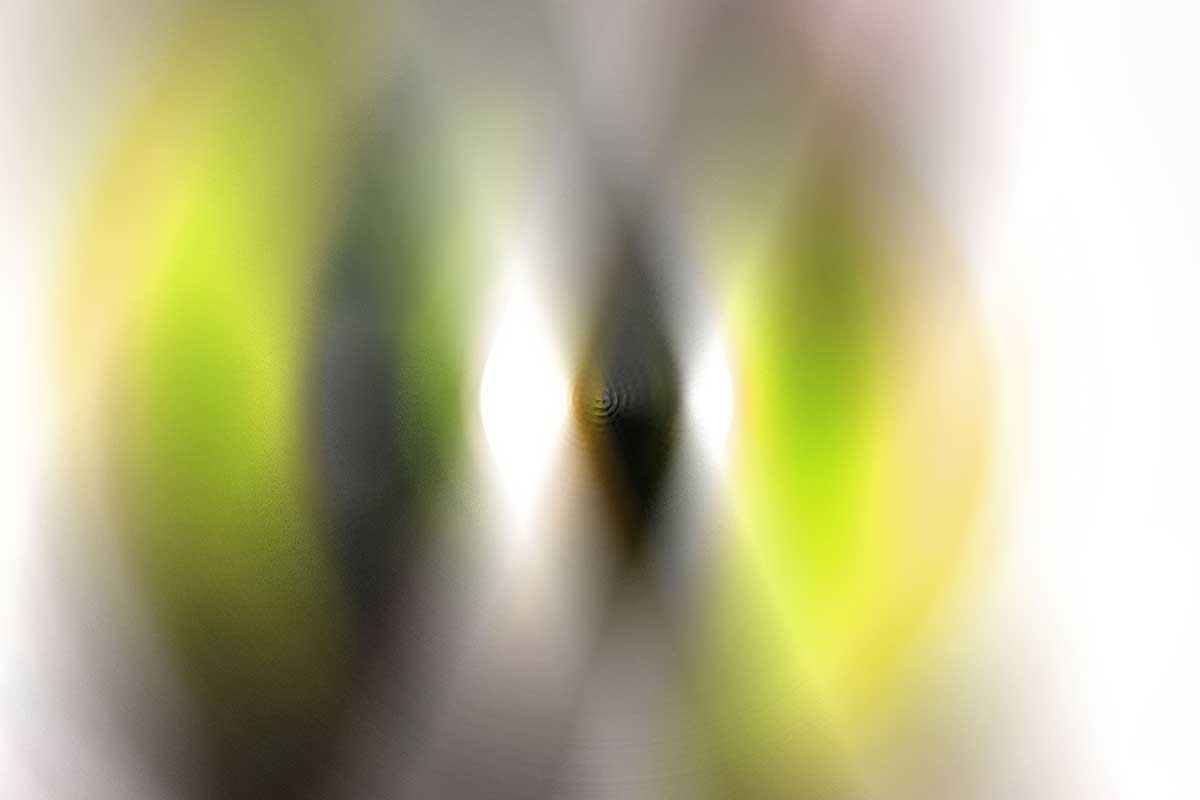
Tim Claxton / Alamy Stock Photo
The theory, by physicist David Bohm, has been resurrected after researchers carried out experiments on photons that seemed to support it.
The behaviour of the quantum world has befuddled physicists for nearly a century. “We have had geniuses working on it and we still have a problem,” says Basil Hiley, a quantum physicist at Birkbeck College at the University of London, who worked with Bohm until the latter’s death in 1992.
The famous illustration is the double-slit experiment: if you fire photons at two slits, our classical intuition expects each to pass through one or the other slit and hit a screen on the other side, making a single mark indicative of its particle nature. But when you try it, the photons create an interference pattern of light and dark bands on the screen, as if each photon behaved like a wave and passed through both slits simultaneously.
The dominant explanation of such behaviour is called the Copenhagen interpretation, which states that the question of whether a photon is a wave or a particle has no meaning until you make a measurement – and then it becomes one or the other depending on which property you measure. The other favoured explanation is the many-worlds interpretation, under which each possible state of the photon becomes manifest in an alternate world.
Underlying order
But in 1952, Bohm suggested that the quantum world only appears weird because we don’t know enough about its underlying reality. Beneath the quantum weirdness, he said, reality is orderly.“It’s a very deterministic description, where all the particles in nature have definite positions and follow definite trajectories,” says Aephraim Steinberg of the University of Toronto in Canada.
Many recent experiments have suggested that no such hidden reality exists. However, they have only ruled out a specific class of theories in which the hidden reality of any particle is local, and not influenced by something far away.
Bohm’s ideas involve non-local hidden reality, in which everything depends on everything. In his universe, something happening in a distant galaxy is influencing you right now and vice versa, however minor the effect.
Take the debate over whether an electron is a wave or a particle. Bohm’s theory says that it’s both: an electron is a particle with a definite trajectory, but this path is governed by a wave upon which the electron rides. The wave can also be influenced by other particles, which in turn changes the trajectory of the electron.
Surrealism
This “Bohmian mechanics” did not go down well with everyone. A 1992 thought experiment seemed to sound the death knell. Called the ESSW experiment after the initials of its four authors, it returned to the double-slit experiment with Bohm in mind.Bohmian mechanics holds that a photon will either go through the top or the bottom slit, not both. Guided by their waves, the top ones will end up at the top half of the screen and the bottom ones at the bottom half.
The ESSW experiment added a twist. The researchers imagined a detector in front of the slits that could tell whether a photon went through the top or bottom one. Their mathematical analysis showed something very strange: a photon could sometimes trigger the top-slit detector, but end up at the bottom half of the screen. They called this forbidden path a “surreal” trajectory, although theorists at the time debated its validity.
Bohm is back
Now Steinberg and colleagues have performed the ESSW experiment themselves – and concluded that Bohm is back in the game.They started with pairs of entangled photons, which are so intimately related that measuring the polarisation state of one affects the polarisation state of the other, no matter how far apart they are. One of each pair was sent into apparatus analogous to the double-slit set-up.
Depending on whether its polarisation was vertical or horizontal, the photon was steered towards either the top or bottom slit. As it traversed the apparatus, the researchers probed its position gently enough to preserve its quantum nature. Each measurement gave only an approximate value, but by tracking a vast ensemble of identical photons, they were able to reconstruct the set of trajectories the photons followed from the slit to the screen.
The other photon of each pair remained outside the double-slit apparatus, allowing the researchers to use it as a probe – analogous to the detector in the ESSW experiment. Because of their entanglement, the probe’s polarisation should dutifully reflect the slit its partner took as it entered the apparatus. And indeed, at the start of the trajectory, the probe photon’s polarisation state accurately mirrored the path its partner took.
The team kept measuring the probe’s photon state as its partner sped through the apparatus, and found that the polarisation kept changing. For example, if it was horizontally polarised to start with, this suggested that its partner went through the bottom slit.
But by the time the partner reached the screen, the probe photon had ended up in a superposition of both states, equally likely to be horizontally or vertically polarised. Depending on when you looked at the probe photon’s state, you either got correct information about its partner’s path, or would be led astray.
Real world
The team thinks this means that the trajectory of the first photon changed the probe’s polarisation – in line with Bohm’s ideas on non-local interactions. This could resolve the problem identified by the ESSW thought experiment. ESSW thought the problem lay with the photon’s trajectory, but Steinberg and his colleagues showed that the trajectory is real – but the detector is an unreliable witness.“I’m happy to see this resolution. It restores my taste for Bohmian mechanics,” says Steinberg. “We want to bring it back to its rightful place among all other interpretations.”
Hiley is impressed by the experiment. “It is a new way of looking at quantum non-locality, which vindicates the Bohm position,” he says.
Sheldon Goldstein, an expert on the foundations of quantum mechanics at Rutgers University in New Jersey, points out that the experiment’s observation of particle trajectories predicted by Bohmian mechanics does not prove that Bohm’s theory on the nature of reality was correct. Such paths can also be explained using other theories, he says.
But Goldstein says there are changes afoot. “After decades and decades, people are taking Bohmian mechanics a little bit more seriously,” he says. “There was a time when you couldn’t even talk about it because it was heretical. It probably still is the kiss of death for a physics career to be actually working on Bohm, but maybe that’s changing.”
Journal reference: Science Advances, DOI: 10.1126/science.1501466
No comments:
Post a Comment